文章信息
- 余再鹏, 万晓华, 胡振宏, 王民煌, 刘瑞强, 郑璐嘉, 何宗明, 黄志群
- YU Zaipeng, WAN Xiaohua, HU Zhenhong, WANG Minhuang, LIU Ruiqiang, ZHENG Lujia, HE Zongming, HUANG Zhiqun
- 亚热带杉木和米老排人工林土壤呼吸对凋落物去除和交换的响应
- Contrasting responses of soil respiration to litter manipulation in subtropical Mytilaria laosensis and Cunninghamia lanceolata plantations
- 生态学报, 2014, 34(10): 2529-2538
- Acta Ecologica Sinica, 2014, 34(10): 2529-2538
- http://dx.doi.org/10.5846/stxb201309092240
-
文章历史
- 收稿日期:2013-9-9
- 网络出版日期:2014-02-20
2. 福建师范大学地理科学学院, 福州 350007;
3. 福建农林大学林学院, 福州 350002
2. College of Geographical Science, Fujian Normal University, Fuzhou 350007, China;
3. College of Forestry, Fujian Agriculture and Forestry University, Fuzhou 350002, China
全球土壤(0—3m)碳储量高达2300 Pg,其中凋落物层碳储量200 Pg[1]。每年土壤呼吸排放通量约为79.3—81.8 Pg C/a,占陆地生态系统呼吸的60%—90%,是化石燃料燃烧释放CO2的10—13倍,其微小波动都会对大气CO2浓度产生显著影响,进而直接影响全球碳平衡[2]。凋落物是土壤碳库的重要组成部分,全球每年土壤呼吸释放CO2中约有50 Pg C来自于凋落物和土壤有机质分解[3]。全球气候变化(降雨、气温和大气CO2浓度变化)引起的森林净初级生产力的增加会显著提高森林凋落物量[4, 5],而凋落物输入数量[5]和质量[6, 7]的变化都可能引起土壤呼吸速率及温度敏感性的改变,不同区域和森林类型去除和添加凋落物的实验为揭示全球变化背景下凋落物对土壤呼吸的影响机制提供了证据。热带大尺度凋落物控制实验表明增加凋落物能显著提高土壤碳的排放速率,凋落物增加30%将导致土壤碳释放量达到0.6 t C hm-2a-1,将部分抵消热带森林土壤净碳吸存[8]。
凋落物对呼吸的贡献主要源于两部分,一是凋落物分解产生的CO2的排放,二是新鲜凋落物的输入刺激土壤中原有有机质的分解,即“激发效应”[9, 10, 11, 12]。众多研究发现去除凋落物能导致土壤呼吸速率相应的降低,大多数是因为移除了地表有效碳源或改变了土壤水热条件[13, 14, 15];而添加凋落物能显著地提高土壤呼吸速率,原因是新鲜凋落物的输入带来了更为有效的底物碳源[13, 14]或是加倍凋落物产生了正激发效应[8, 12]。此外,添加和去除凋落物也会显著影响土壤中的微生物生物量和活性,进而影响微生物异养呼吸速率[16, 17]。添加和去除凋落物对土壤呼吸的影响既有短期效应[14, 18],也会持续多年[5, 12],并且会因森林类型[19, 20]、土地利用方式[21]以及气候的干湿季节变化而异[19]。国内关于森林凋落物呼吸的研究有油松林[15]、枫香、樟树[22]、杉木[18]、马尾松[23]、刺槐[24]等,大多是讨论添加和去除凋落物对土壤呼吸的影响,而关于不同树种凋落物对土壤呼吸的影响的少见报道。
本研究以亚热带杉木人工林采伐迹地上营造的米老排(Mytilaria laosensis)和杉木(Cunninghamia lanceolata)人工林作为对比研究样地,通过去除和互换凋落物的野外控制实验,对比不同树种凋落物对土壤呼吸的影响及其贡献,揭示不同数量和质量凋落物对土壤呼吸的影响机制,为准确评估不同树种凋落物对土壤呼吸的贡献及人工林碳吸存潜力提供参考。
1 材料与方法 1.1 试验地概况及实验设计试验地设在福建省南平市峡阳国有林场(26°48′N,117°59′E),选取杉木林采伐迹地上营造的19年生米老排和杉木两个人工林为研究对象。试验地海拔229—246 m,坡度15°—30°。所在区域属中亚热带湿润季风气候,年均温20.0 ℃,雨量充沛,全年各季节均有降雨,年均降水量为1653 mm。土壤为石英黑云母片岩发育的山地红壤,表层疏松,土层深厚。2011年7月调查时,米老排人工林林下植被主要有苦竹(Pleioblastus amarus)、芒萁(Dicranopteris dichotoma)、狗脊(Woodwardia japonica)、玉叶金花(Mussaenda pubescens)、杜茎山(Maesa japonica)等,数量较少;杉木人工林林下植被有观音座莲(Angiopteris fokiensis)、芒萁、粗叶榕(Ficus simplicissima)、稀羽鳞毛蕨(Dryopteris sparsa)、江南卷柏(Selaginella moellendorffii)、淡竹叶(Lophatherum gracile)、苦竹、狗脊等,林下植被较多。两种林分基本情况见表1 。
林分类型 Forest type | 土壤容重 Bulk density /(g/cm3) | 土壤 pH | 土壤全碳储量 Soil total C /(t/hm2) | 土壤全氮储量 Soil total N /(t/hm2) | 土壤碳氮比 Soil C/N ratio | 郁闭度 Canopy density | 平均树高 Tree height/m | 平均胸径 DBH/cm |
数值为平均值±标准差; 同一行不同小写字母表示两种林分之间差异显著(P<0.05) | ||||||||
米老排M.laosensis | 1.08±0.10a | 4.3±0.2a | 56.7±12.1a | 3.7±0.8a | 16.9±0.4a | 0.9 | 15.4±2.1a | 14.7±2.8a |
杉木C.lanceolata | 1.10±0.18a | 4.6±0.3a | 42.5±4.9a | 3.0±0.5a | 15.7±1.9a | 0.7 | 13.8±3.5a | 15.9±3.6a |
林分类型 Forest type | 年凋落物量 Litter biomass/(t/hm2) | 凋落物碳含量 Litter C/(mg/g) | 凋落物氮含量 Litter N /(mg/g) | 凋落物碳氮比 Litter C/N /ratio | 凋落物层现存量 Forest floor biomass/(t/hm2) | 凋落物层碳氮比 Forest floor C/N ratio | 细根生物量 Fine root biomass/(g/m2) | |
米老排M.laosensis | 9.81±1.73a | 469.3±11.0a | 19.3±1.5a | 24.4±1.2a | 7.90±2.0a | 55.8±7.1a | 981±360a | |
杉木C.lanceolata | 4.96±0.9b | 513.6±7.8b | 8.9±0.6b | 57.7±2.3b | 4.94±1.33b | 59.5±12a | 767±172a |
2011年11月,在两种人工林中分别采用裂区设计方法设置4个区组,每个区组随机分布A、 B、C 3种处理。每个处理设立大小为2 m×2 m的小区,两种林分各设置12个小区。小区具体处理方法为(A)去除凋落物,剔除小区内地面表层全部枯落物,并在小区上方1 m处放置2.5 m×2.5 m的尼龙网(1 mm×1 mm网眼),以截留并阻止凋落物落入小区内;(B)凋落物互换,将米老排和杉木林凋落物等面积交换,即将杉木林中去除凋落物处理小区内尼龙网上截留的凋落物转移到米老排小区内,而米老排小区内的凋落物转移到杉木林小区内。每月定期交换1次;(C)对照,即不做任何处理,保留小区原有状态作为参考。
1.2 土壤呼吸及其相关环境因子的测定每个小区中心位置埋设1个内径20.0 cm、高10.0 cm的PVC材质的土壤呼吸圈,圈底部埋入土壤5 cm深,埋入地下部分留有直径为0.5 cm的4个圆孔,以加强环内外土壤水热交换,测量期间呼吸圈位置保持不变[25]。从2012年1月至2013年2月,利用Li-8100开路式土壤碳通量观测系统(LI-8100,Li-Cor公司,美国)测定土壤呼吸速率。根据本区域Sheng等[26]相关研究结果,在每月中旬选择2—3d晴朗少雨的9:00—12:00测定土壤呼吸速率,每个土壤环测量时间为2 min。同时,采用数字式瞬时温度计(AM-11T,Avalon公司,美国)测定呼吸圈附近地下10 cm处的土壤温度,采用时域反射仪(TDR)(Model TDR300,Spectrum公司,美国)测定呼吸圈附近地下0—12 cm处土壤体积含水量,每个呼吸圈周围测量3次取平均值。为减少TDR测量误差,在实验室内采用烘干法建立实测值(W)与烘干值(W0)之间的一元线性方程W=0.735W0+7.397 (R2=0.896)来校正TDR实测值[25]。
1.3 土壤易变性有机碳及微生物的测定在观测期间,每月定期用内径为2 cm的PVC管在每个小区内取0—10 cm土样,用冰盒冷藏带回实验室用于测定可溶性有机碳(DOC)和微生物生物量碳(MBC)含量。DOC含量采用冷水浸提[27],MBC采用氯仿-熏蒸法浸提[28]。用总有机碳分析仪(TOC-VCPH/CPN analyzer,日本)测定浸提液中的有机碳含量。此外,在2012年5月采集各小区0—5 cm土样用磷脂脂肪酸(PLFA)方法测定土壤中的微生物群落组成[29]。
1.4 数据处理方法分别采用线性与非线性模型拟合土壤呼吸速率与土壤温度和土壤含水量的关系,回归模型如下:
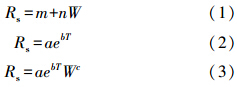
式中,Rs为土壤呼吸速率(μmol CO2 m-2s-1),T为地下10 cm处土壤温度,W为地下0—12 cm土壤含水量,a,b,c,m和 n为待定参数。

式中,b为(2)式中计算的常量。
土壤呼吸年通量:以每月测定的土壤呼吸速率代表该月的平均值,乘以各月时间后累计相加即可算出年通量。
激发效应[30]:
交换凋落物的激发效应=(林分a交换凋落物小区土壤呼吸年通量-林分a去除凋落物小区土壤呼吸年通量)-林分b年凋落物量×C%×同期b凋落物在林分a中的年分解速率
式中,a代表米老排(或杉木林),b代表杉木林(或米老排);C%为凋落物碳含量;凋落物年分解速率为同期米老排和杉木两种凋落物交换分解(网袋法)计算得出的凋落物分解系数。
采用重复测量方差(Repeated-measures ANOVA)分析两种林分内不同处理土壤呼吸、土壤温度和土壤含水量的差异。用单因素方差分析两种林分不同处理小区平均土壤呼吸速率、碳排放通量、DOC、MBC以及微生物生物量的差异。
2 结果与分析 2.1 土壤呼吸、温度和湿度的季节动态两种林分不同处理的土壤呼吸速率均有显著的季节变化,呈现出单峰曲线趋势(图1,P值均为0.000)。米老排人工林3种处理的土壤呼吸速率在1月至6月快速上升,到6月份出现最高值,之后缓慢下降到1月进入最低值。杉木人工林去除凋落物和对照处理的土壤呼吸速率在6月出现最高值,之后缓慢下降到1月份进入最低值,而交换凋落物的土壤呼吸速率在6月份上升到较高值后一直保持到9月份,之后缓慢下降进入低值。米老排人工林去除凋落物和交换凋落物的土壤呼吸速率的平均值分别为(1.79±0.29)和(2.18±0.18) μmol CO2·m-2 s-1,显著低于对照(2.45±0.19) μmol CO2·m-2 s-1(P<0.01)。杉木林人工林去除凋落物和对照的土壤呼吸速率的的平均值分别为(1.77±0.28) 和(1.90±0.13) μmol CO2·m-2 s-1,而交换凋落物的平均值为(2.29±0.33) μmol CO2·m-2 s-1,显著高于对照(P=0.004)。
米老排和杉木林土壤温度和含水量均有显著的季节变化(图1,P值均<0.001)。在同一林分内,不同处理的土壤温度和土壤含水量均无显著差异(P值均>0.05)。月份与土壤温度和含水量之间均不存在交互作用(P>0.05)。两种林分间,不同处理土壤温度无显著差异,土壤含水量的差异均达到显著水平(P值均<0.001),且均为杉木人工林高于米老排人工林。米老排不同处理土壤含水量的平均值在25.1%—25.8%之间,杉木林为27.0%—28.0%。
2.2 土壤呼吸的年通量在两种林分中,土壤呼吸年通量对凋落物去除和交换的响应不同(表2)。米老排人工林,去除凋落物处理相比对照处理显著降低了土壤呼吸年通量,降低幅度为29.8%;交换凋落物则显著降低14.2%。杉木人工林去除凋落物处理土壤碳排放通量减少6.1%,但差异不显著(P>0.05);而交换凋落物则使土壤呼吸年通量显著增加37.8%。米老排和杉木林凋落物年碳输入量分别为472 g C·m-2a-1和247 g C·m-2a-1。利用凋落物(叶)分解系数计算的由凋落物交换引起的土壤碳排放的增加量分别为米老排人工林86 g C·m-2a-1,杉木人工林149 g C·m-2a-1,占年通量的比例分别为米老排林9.8%,杉木林16.7%。
![]() |
图 1 两种林分不同凋落物处理的土壤呼吸速率、温度和含水量的季节动态 Fig. 1 Seasonal dynamics of soil respiration,soil temperature and volumetric soil water content under M. laosensis and C.lanceolata plantations (mean±SD,n=4) |
林分 Forest stand | 年通量 Annual fluxes | 改变百分比 % of change | 激发效应 Priming effect | ||||
去除凋落物 Litter exclusion/ (g C·m-2·a-1) | 交换凋落物 Litter transplant/ (g C·m-2·a-1) | 对照处理 Control/ (g C·m-2·a-1) | 概率 P | 去除凋落物 Litter exclusion/% | 交换凋落物 Litter transplant/% | 交换凋落物 Litter transplant/(g C·m-2·a-1) | |
同一行不同小写字母不同处理间差异显著 | |||||||
米老排M. laosensis | 725±117a | 880±91b | 1027±88c | 0.006 | -29.8±5.4 | -14.2±7.8 | 86 |
杉木C. lanceolata | 609±11.6a | 893±136b | 649±48a | 0.016 | -6.1±8.2 | 37.8±17.8 | 149 |
两种林分不同凋落物处理的土壤呼吸速率均与土壤温度有较好的指数关系(表3),而与土壤湿度呈负相关,且在米老排人工林中达到显著水平(P<0.05)。采用土壤温度和湿度的双因素模型能分别解释米老排人工林中去除凋落物、交换和对照处理土壤呼吸的68.9%、77.0%和69.6%;杉木人工林中的53.0%、36.2%和63.8%。米老排人工林中去除和交换凋落物均降低了土壤呼吸的温度敏感性Q10值,而杉木林中仅去除凋落物处理降低了土壤呼吸的Q10值。
处理 | Rs = m+nW | Rs=aebT | Rs =aebT Wc | Q10 | ||||||||
m | n | R2 | a | b | R2 | a | b | c | R2 | |||
* P<0.05; **P<0.001; ns: not significant | ||||||||||||
米老排 | 去除凋落物Litter exclusion | 3.103 | -0.052 | 0.088* | 0.596 | 0.062 | 0.678** | 0.244 | 0.068 | 0.274 | 0.689** | 1.86 |
交换凋落物Litter transplant | 4.036 | -0.073 | 0.104* | 0.593 | 0.072 | 0.755** | 0.152 | 0.081 | 0.374 | 0.770** | 2.05 | |
对照Control | 4.734 | -0.089 | 0.101* | 0.624 | 0.076 | 0.670** | 0.089 | 0.088 | 0.531 | 0.696** | 2.14 | |
杉木 | 去除凋落物Litter exclusion | 2.488 | -0.026 | 0.024 ns | 0.997 | 0.034 | 0.530** | 1.034 | 0.034 | -0.010 | 0.530** | 1.40 |
交换凋落物Litter transplant | 3.777 | -0.059 | 0.07* | 1.080 | 0.042 | 0.339** | 3.461 | 0.036 | -0.323 | 0.362** | 1.52 | |
对照Control | 2.035 | -0.012 | 0.005 ns | 0.848 | 0.042 | 0.599** | 0.200 | 0.047 | 0.411 | 0.638** | 1.52 |
同一处理,米老排人工林土壤DOC和MBC含量均显著高于杉木人工林;但同一林分内,不同处理间土壤DOC和MBC的差异均未达到显著水平(表4)。磷脂脂肪酸分析结果显示,两种林分间土壤微生物总PLFA生物量差异不显著。与对照相比,米老排交换凋落物小区土壤总PLFA生物量显著降低了52%,而杉木林交换凋落物小区土壤总PLFA生物量增加25%。
处理 | 米老排M. laosensis | 杉木C. lanceolata | ||||
可溶性有机碳 | 微生物生物量碳 | 微生物生物量 | 可溶性有机碳 | 微生物生物量碳 | 微生物生物量 | |
同一列不同小写字母表示不同处理间差异显著,不同大写字母表示两种林分间差异显著 (α= 0.05) | ||||||
去除凋落物Litter exclusion | 94±15aA | 756±119aA | 16.4±3.8aA | 29±2aB | 502±64aB | 12.8±2.6aA |
交换凋落物Litter transplant | 86±27aA | 634±137aA | 10.8±1.8bA | 40±13aB | 514±51aB | 12.4±0.6aA |
对照Control | 99±22aA | 722±185aA | 14.6±3.7aA | 32 ±8aB | 437±74aB | 9.9±2.0aA |
相关分析发现,两种林分不同处理的平均土壤呼吸速率与土壤年均DOC及MBC含量均显著正相关(图2)。
![]() |
图 2 两种林分不同处理平均土壤呼吸速率与微生物生物量碳和可溶性有机碳的关系(n=24) Fig. 2 Relationships between average soil respiration rate and microbial biomass carbon or dissolved organic carbon concentration with different treatments under two tree species (n=24) |
交换凋落物和对照处理小区5月份土壤呼吸速率及2012年年均土壤呼吸速率与一些主要微生物PLFA含量及真菌细菌比显著相关(表5)。
18:1 w7c | 18:1 w5c | a17:0 | 16:1 w5c | 10Me 17:0 | 18:1 w9c | 18:2 w6,9c | 真菌总数 | 真菌:细菌 | ||
表中只列出了相关性显著的PLFA[29] | ||||||||||
5月份土壤呼吸速率 | R2 | 0.529 | - | - | 0.640 | 0.523 | 0.601 | 0.737 | 0.679 | 0.561 |
P | 0.043 | - | - | 0.010 | 0.046 | 0.018 | 0.002 | 0.005 | 0.029 | |
2012年均呼吸速率 | R2 | 0.518 | 0.432 | 0.512 | 0.629 | - | 0.599 | 0.687 | 0.653 | 0.658 |
P | 0.040 | 0.094 | 0.043 | 0.009 | - | 0.014 | 0.003 | 0.006 | 0.006 |
通常,去除或添加凋落物会显著降低或提高土壤呼吸速率,而土壤呼吸速率降低或增加的幅度受地区(温带[31]、亚热带[14, 32]、热带[8, 10])、森林类型[5, 7]以及实验延续时间[4, 15, 31]的影响。本研究中,去除凋落物处理使米老排人工林土壤呼吸年通量显著减少29.8%,与亚热带阔叶树种去除凋落物使土壤呼吸显著降低的结论一致[20, 22]。杉木人工林中去除凋落物小区土壤呼吸年通量仅降低6.1% (P>0.05),低于湖南会同地区杉木人工林去除凋落物土壤呼吸速率降低15.0%—22.6%的幅度[14, 18]。这种差异可能与研究区域、处理延续的时间以及开始测量呼吸的时间不同有关[13, 14]。米老排和杉木凋落物互换后,杉木小区土壤呼吸年通量提高37.8%,而米老排小区则降低了14.2%,这说明不同数量和质量凋落物对土壤呼吸的影响存在显著差异,也与Bréchet 等[33]研究发现的不同树种凋落物对土壤呼吸有显著影响的结论一致。本研究区域,米老排凋落物年归还量接近杉木林的2倍,质量较高(C/N低,Lignin/N低),容易分解淋溶转化成可溶性有机碳,为微生物异养呼吸提供大量有效碳源[29];而杉木(针叶)凋落物木质素化合物含量较高,分解较慢,易形成酸性粗腐殖质可能抑制土壤微生物活性,进而降低土壤呼吸速率[34]。
众多研究表明,添加凋落物能激发并加快土壤中原有机质的分解,从而大幅提高土壤呼吸速率[5, 8, 10]。添加凋落物产生的激发效应可达到11.5%—21.6%[12]。本研究中,交换凋落物相对于去除凋落物处理产生了不同程度的激发效应(表2)。虽然不能排除由凋落物输入而导致地下根系及菌根的响应而增加的土壤呼吸[10],然而米老排凋落物在杉木林次小区中的激发效应(16.7%)大于杉木凋落物在米老排次小区中的激发效应(9.8%)。这可能与单位面积上米老排凋落物较大的生物量输入的较多的碳有关;另外,米老排(阔叶)凋落物质量高,养分释放比杉木凋落物快,可能更容易激发土壤中原有有机碳矿化[35]。两种林分中凋落物产生的激发效应显著地影响了土壤呼吸通量,预示着未来森林地上净生产力的增加将会显著地影响土壤地下碳库[30]。
土壤温度和湿度是影响土壤呼吸最重要的环境因子,通过调节植物光合作用及根系生长代谢而影响土壤呼吸[36, 37]。本研究中米老排和杉木人工林小区土壤呼吸速率与土壤温度有显著的指数关系,而与土壤含水量负相关,表明较高的土壤湿度可能对土壤呼吸有一定抑制作用[14],而土壤温度是驱动土壤呼吸最重要的环境因子(表3)。但Wang等[18]研究的杉木人工林不同凋落物处理土壤呼吸速率与土壤湿度无显著相关性,这可能与研究期间降雨量和土壤含水量的差异有关[18]。去除或添加凋落物处理通常会破坏或加强凋落物层对表层土壤的保护[19, 24],影响土壤表层温度和湿度[15],进而影响土壤呼吸速率。本研究中,不同凋落物处理对土壤温度和湿度的影响均不显著,且去除和交换凋落物导致的土壤呼吸的改变量与土壤温度和湿度的改变量无显著相关性(P>0.05),表明由不同凋落物处理引起的土壤温度和湿度的变化不足以影响土壤呼吸速率[18]。整个观测期间降雨量大,土壤湿度保持在较高水平 (图1),不利于土壤CO2的扩散和排放[38]。
凋落物作为地上部分重要的碳源,其淋溶或分解产生的易变性有机碳是微生物异养呼吸的重要碳源[39, 40]。去除和添加凋落物通常会引起可溶性有机碳的改变[12, 13, 41]。本研究中,虽然不同凋落物处理对土壤DOC含量无显著影响,但两种林分不同处理DOC含量与土壤呼吸速率显著正相关,这与Cleveland等[42]研究的由凋落物输入的可溶性有机碳与土壤呼吸速率显著相关的结论一致。此外,新鲜凋落物淋溶带来的DOC可能刺激土壤中原有有机质的矿化,提高土壤呼吸速率[9, 10, 11]。本研究中两种凋落物均产生了正激发效应,导致次小区土壤呼吸速率增加的幅度高于凋落物分解自身产生的呼吸的量。凋落物交换导致杉木林土壤呼吸的大幅升高可能与高质量的米老排淋溶带来的大量的DOC,刺激了土壤中原有机碳的矿化有关[8, 11];同时,随着碳矿化速率的提高,土壤中CO2浓度升高,这也可能导致根系及菌根生物量增加,进而增加根系和菌根呼吸速率;另外,由凋落物淋溶带来的氮、磷等养分提高了微生物分解底物的有效性,也会影响土壤呼吸速率[10]。
已有研究发现,去除或添加凋落物会显著影响土壤微生物生物量和活性,导致土壤呼吸速率发生变化[16, 17]。本研究中,交换凋落物处理导致米老排小区土壤MBC含量以及微生物总PLFA生物量均有不同程度减少,而在杉木人工林不同程度增加[29],这与Ushio等[40]发现的不同树种(针叶和阔叶)对土壤微生物生物量具有显著影响结论一致。也与Subke等[44]发现的土壤微生物添加高质量的凋落物能显著提高土壤中微生物生物量和活性的结论一致。Wang等[14]研究表明,杉木人工林中不同碳输入处理的土壤呼吸与微生物PLFA含量显著相关。本研究中两种林分交换凋落物和对照处理的土壤呼吸速率与主要的微生物PLFA含量显著相关,表明凋落物交换引起的土壤微生物生物量的改变显著影响了土壤呼吸速率。值得注意的是,土壤微生物群落组成具有较强的时间变异[45],本研究中土壤PLFA只有一次取样,不能完全反映微生物群落的动态变化,因而仅分析土壤呼吸与微生物生物量的关系。
研究表明,土壤呼吸的温度敏感性受到土壤生物、底物质量和底物供应等多种因素的显著调控,且会随着土壤水分、温度的变异而发生变化[46]。本研究中,米老排小区去除和交换凋落物处理均导致土壤呼吸的温度敏感性Q10值降低,这与Boone 等[30]研究结果一致,而与王光军等[18]研究结果相反,表明在不同地区、不同森林类型以及不同观测时段,土壤呼吸对凋落物处理的响应存在差异。杉木人工林次小区Q10显著低于Zheng等[47]研究的我国亚热带森林Q10值的变化范围(1.56—2.39),而与陈光水等[48]报道的我国森林土壤呼吸的Q10值范围在1.33—5.53之间的结论一致。
4 结论亚热带人工林不同凋落物处理对土壤呼吸的影响及贡献因树种而异。去除和交换凋落物导致米老排人工林CO2年排放量显著减少29.8%和14.2%,杉木人工林则分别减少6.1%和增加37.8%。两种林分交换凋落物处理产生了不同程度的激发效应,米老排凋落物相对于杉木凋落物具有更大的激发效应。两种林分不同凋落物处理土壤呼吸速率与土壤可溶性有机碳含量、微生物生物量碳含量以及主要微生物PLFA显著相关。
致谢: 感谢福建省南平市峡阳国有林场翁贤权等同志对野外试验样地的维护和野外工作的大力支持!
[1] | Davidson E A, Janssens I A. Temperature sensitivity of soil carbon decomposition and feedbacks to climate change. Nature, 2006, 440 (7081): 165-173. |
[2] | Peng S S, Piao S L, Wang T, Sun J Y, Shen Z H. Temperature sensitivity of soil respiration in different ecosystems in China. Soil Biology and Biochemistry, 2009, 41 (5): 1008-1014. |
[3] | Raich J W, Potter C S, Bhagawati D. Interannual variability in global soil respiration, 1980-94. Global Change Biology, 2002, 8: 800-812. |
[4] | Xu S, Liu L, Sayer E J. Variability of aboveground litter inputs alters soil physicochemical and biological processes: a meta-analysis of litterfall-manipulation experiments. Biogeosciences Discussions, 2013, 10 (3): 5245-5272. |
[5] | Sayer E J. Using experimental manipulation to assess the roles of leaf litter in the functioning of forest ecosystems. Biological Reviews of the Cambridge Philosophical Society, 2006, 81 (1): 1-31. |
[6] | Liu L L, King J S, Giardina C P. Effects of elevated concentrations of atmospheric CO2 and tropospheric O3 on leaf litter production and chemistry in trembling aspen and paper birch communities. Tree Physiology, 2005, 25: 1511-1522. |
[7] | Kaneda S, Frouz J, Baldrian P, Cajthaml T, Krišt fek V. Does the addition of leaf litter affect soil respiration in the same way as addition of macrofauna excrements (of Bibio marci Diptera larvae) produced from the same litter? Applied Soil Ecology, 2013, 72: 7-13. |
[8] | Sayer E J, Heard M S, Grant H K, Marthews T R, Tanner E V J. Soil carbon release enhanced by increased tropical forest litterfall. Nature Climate Change, 2011, 1: 304-307. |
[9] | Schaefer D A, Feng W, Zou X. Plant carbon inputs and environmental factors strongly affect soil respiration in a subtropical forest of southwestern China. Soil Biology and Biochemistry, 2009, 41 (5): 1000-1007. |
[10] | Kuzyakov Y. Priming effects Interactions between living and de ad organic matter. Soil Biology and Biochemistry, 2010, 42: 1363-1371. |
[11] | Wang Q K. Responses of forest soil carbon pool and carbon cycle to the changes of carbon input. Chinese Journal of Applied Ecology, 2011, 22 (4): 1075-1081. |
[12] | Crow S E, Lajtha K, Bowden R D, Yano Y, Brant J B, Caldwell B A, Sulzman E W. Increased coniferous needle inputs accelerate decomposition of soil carbon in an old-growth forest. Forest Ecology and Management, 2009, 258 (10): 2224-2232. |
[13] | Leff J W, Wieder W R, Taylor P G, Townsend A R, Nemergut D R, Grandy A S, Cleveland C C. Experimental litterfall manipulation drives large and rapid changes in soil carbon cycling in a wet tropical forest. Global Change Biology, 2012, 18 (9): 2969-2979. |
[14] | Wang Q K, He T X, Wang S L, Liu L. Carbon input manipulation affects soil respiration and microbial community composition in a subtropical coniferous forest. Agricultural and Forest Meteorology, 2013, 178-179: 152-160. |
[15] | Wang J S, Zhao X H, Zhang C Y, Kang F F, Shang B S, Chi Q J. Changes of carbon input influence soil respiration in a Pinus tabulaeformis plantation. Acta Ecologica Sinica, 2012, 32 (9): 2768-2777. |
[16] | Li Y Q, Xu M, Sun O J, Cui W C. Effects of root and litter exclusion on soil CO2 efflux and microbial biomass in wet tropical forests. Soil Biology and Biochemistry, 2004, 36 (12): 2111-2114. |
[17] | Brant J B, Sulzman E W, Myrold D D. Microbial community utilization of added carbon substrates in response to long-term carbon input manipulation. Soil Biology and Biochemistry, 2006, 38 (8): 2219-2232. |
[18] | Wang G J, Tian D L, Yan W D, Zhu F, Xiang W H, Liang X C. Effects of aboveground litter exclusion and addition on soil respiration in a Cunninghamia lanceolata plantation in china. Chinese Journal of Plant Ecology, 2009a, 33 (4): 739-747. |
[19] | Deng Q, Liu S Z, Liu J X, Meng Z, Zhang D Q. Contributions of litter-fall to soil respiration and its affacting factors in southern subtropical forests of China, Advances in Earth Science, 2007(22): 976-986 |
[20] | Yan W, Chen X, Tian D, Peng Y, Wang G, Zheng W. Impacts of changed litter inputs on soil CO2 efflux in three forest types in central south China. Chinese Science Bulletin, 2012, 58 (7): 750-757. |
[21] | Wang L L, Song C C, Guo Y D, Liu D Y, Yang G S. Contribution of litter to soil respiration under different land-use types in Sanjiang Plain. Environmental Science, 2009, 30 (11): 3130-3135. |
[22] | Wang G J, Tian D L, Yan W D, Zhu F, Li S Z. Impact of litter addition and exclusion on soil respiration in a Liquidambar formosana forest and a nearby Cinnamomum camphora forest of central southern China. Acta Ecologica Sinica, 2009b, 29 (2): 643-652. |
[23] | Wang G J, Tian D L, Yan W D, Zhu F, Li S Z. Response of soil respirations to litterfall exclusion and addition in Pinus massoniana plantation in Hunan, China. Scientia Silvae Sinicae, 2009c, 45 (1): 27-30. |
[24] | Zhou X G, Guo S L, Che S G, Zhang F, Zou J L, Zhang Y J, Nan Y F, Li Z, Pu H. Aboveground litter contribution to soil respiration in a black locust plantation in the Loess Plateau. Acta Ecologica Sinica, 2012, 32 (7): 2150-2157. |
[25] | Hu Z H, Fan S H, Huang Z Q, He Z M, Yu Z P, Wang M H, Weng X Q. Effects of harvest residue management on soil respiration of Chinese fir plantations. Scientia Silvae Sinicae, 2013, 49(5): 25-29 |
[26] | Sheng H, Yang Y S, Yang Z J, Chen G S, Xie J S, Guo J F, Zou S Q. The dynamic response of soil respiration to land-use in subtropical China. Global Change Biology, 2009, 16 (2): 1107-1121. |
[27] | Curtin D, Wright C E, Beare M H, Mccallum F. M. Hot water-extractable nitrogen as an indicator of soil nitrogen availability. Soil Science Society of America Journal, 2006, 70 (5): 1512-1521. |
[28] | Joergensen R G. The fumigation-extraction method to estimate soil microbial biomass: Calibration of the kEC value. Soil Biology and Biochemistry, 1996,28(1): 25-31. |
[29] | Huang Z Q, Wan X H, He Z M, Yu Z P, Wang M H, Hu Z H, Yang Y S. Soil microbial biomass, community composition and soil nitrogen cycling in relation to tree species in subtropical China. Soil Biology and Biochemistry, 2013, 62: 68-75. |
[30] | Sulzman E W, Brant J B, Bowden R D, Lajtha K. Contribution of aboveground litter, belowground litter, and rhizosphere respiration to total soil CO2 efflux in an old growth coniferous forest. Biogeochemistry, 2005, 73 (1): 231-256. |
[31] | Boone R D, Nadelhoffer K J, Canary J D,Kaye J P. Roots exert a strong influence on the temperature sensitivity of soil respiration. Nature, 1998, 396: 570-572. |
[32] | Wang Q K, Liu S, Wang S. Debris manipulation alters soil CO2 efflux in a subtropical plantation forest. Geoderma, 2013, 192: 316-322. |
[33] | Bréchet L, Ponton S, Roy J, Freycon V, Coteaux M M, Bonal D,Epron D. Do tree species characteristics influence soil respiration in tropical forests? A test based on 16 tree species planted in monospecific plots. Plant and Soil, 2009, 319 (1/2): 235-246. |
[34] | Leckie S E,Prescott C E,Grayston S J. Forest floor microbial community response to tree species and fertilization of regenerating coniferous forests. Canadian Journal of Forest Research, 2004, 34(7): 1426-1435. |
[35] | Zhang W D, Wang S L. Effects of NH4+ and NO3- on litter and soil organic carbon decomposition in a Chinese fir plantation forest in South China. Soil Biology and Biochemistry, 2012, 47: 116-122. |
[36] | Luo Y Q, Zhou X H. Soil Respiration and the Environment. London: Academic Press, 2006: 79-92. |
[37] | Moyano F E, Kutsch W L, Rebmann C. Soil respiration fluxes in relation to photosynthetic activity in broad-leaf and needle-leaf forest stands. Agricultural and Forest Meteorology, 2008, 148 (1): 135-143. |
[38] | Luan J W, Liu S R, Zhu X L, Wang J X, Liu K. Roles of biotic and abiotic variables in determining spatial variation of soil respiration in secondary oak and planted pine forests. Soil Biology and Biochemistry, 2012, 44 (1): 143-150. |
[39] | Cleveland C C, Nemergut D R, Schmidt S K,Townsend A R. Increases in soil respiration following labile carbon additions linked to rapid shifts in soil microbial community composition. Biogeochemistry, 2006, 82 (3): 229-240. |
[40] | Laik R, Kumar K, Das D K, Chaturvedi O P. Labile soil organic matter pools in a calciorthent after 18 years of afforestation by different plantations. Applied Soil Ecology, 2009, 42 (2): 71-78. |
[41] | Park J H, Matzner E. Controls on the release of dissolved organic carbon and nitrogen from a deciduous forest floor investigated by manipulations of aboveground litter inputs and water flux. Biogeochemistry, 2003, 66: 265-286. |
[42] | Cleveland C C, Reed S C, Townsend A R. Nutrient regulation of organic matter decomposition in a tropical rain forest.Ecology, 2006, 87: 492- 503. |
[43] | Ushio M, Wagai R, Balser T C, Kitayama K Variations in the soil microbial community composition of a tropical montane forest ecosystem: Does tree species matter? .Soil Biology and Biochemistry, 2008, 40 (10): 2699-2702. |
[44] | Subke J A, Hahn V, Battipaglia G, Linder S, Buchmann N, Cotrufo M F. Feedback interactions between needle litter decomposition and rhizosphere activity. Oecologia, 2004, 139 (4): 551-559. |
[45] | Landesman W J, Dighton J. Response of soil microbial communities and the production of plant-available nitrogen to a two-year rainfall manipulation in the New Jersey Pinelands. Soil Biology and Biochemistry, 2010, 42 (10): 1751-1758. |
[46] | Yang Q P, Xu M, Liu H S, Wang J S, Liu L X, Cui Y G, Zheng Y P. Impact factors and uncertainties of the temperature sensitivity of soil respiration. Acta Ecologica Sinica, 2011, 31 (8): 2301-2311. |
[47] | Zheng Z M, Yu G R, Fu Y L, Wang Y S, Sun X M, Wang Y H. Temperature sensitivity of soil respiration is affected by prevailing climatic conditions and soil organic carbon content: A trans-China based case study. Soil Biology and Biochemistry, 2009, 41 (7): 1531-1540. |
[48] | Chen G S, Yang Y S, Lü P P, Zhang Y P, Qian X L. Regional patterns of soil respiration in China's forests. Acta Ecologica Sinica, 2008, 28 (4): 1748-1761. |
[11] | 王清奎.碳输入方式对森林土壤碳库和碳循环的影响研究进展.应用生态学报,2011,22(4):1075-1081. |
[15] | 汪金松,赵秀海,张春雨,康峰峰,尚宝山,池青俊.改变C源输入对油松人工林土壤呼吸的影响.生态学报,2012,32(9):2768-2777. |
[18] | 王光军,田大伦,闫文德,朱凡,项文化,梁小翠.改变凋落物输入对杉木人工林土壤呼吸的短期影响.植物生态学报2009,33(4):739-747. |
[19] | 邓琦,刘世忠,刘菊秀,孟泽,张德强.南亚热带森林凋落物对土壤呼吸的贡献及其影响因素.地球科学进展,2007(22):976-986 |
[21] | 王丽丽,宋长春,郭跃东,刘德燕,杨桂生.三江平原不同土地利用方式下凋落物对土壤呼吸的贡献.环境科学,2009,30(11):3130-3135. |
[22] | 王光军,田大伦,闫文德,朱凡,李树战.去除和添加凋落物对枫香(Liquidambar formosana)和樟树(Cinnamomum camphora)林土壤呼吸的影响.生态学报,2009b,29(2):643-652. |
[23] | 王光军,田大伦,闫文德,朱凡,李树战.马尾松林土壤呼吸对去除和添加凋落物处理的响应.林业科学,2009c,45(1):27-30. |
[24] | 周小刚,郭胜利,车升国,张芳,邹俊亮,张彦军,南雅芳,李泽,蒲辉.黄土高原刺槐人工林地表凋落物对土壤呼吸的贡献.生态学报,2012,32(7):2150-2157. |
[25] | 胡振宏,范少辉,黄志群,何宗明,余再鹏,王民煌,翁贤权.采伐剩余物管理措施对杉木人工林土壤呼吸的影响.林业科学,2013,49(5):25-29 |
[46] | 杨庆朋,徐明,刘洪升,王劲松,刘丽香,迟永刚,郑云普.土壤呼吸温度敏感性的影响因素和不确定性.生态学报,2011,31(8):2301-2311. |
[48] | 陈光水杨玉盛,吕萍萍,张亿萍,钱小兰.中国森林土壤呼吸模式.生态学报,2008,28(4):1748-1761 |